3 Study Area and Data
3.1 Study Area
The Canary Islands archipelago is formed by eight populated islands and six islets of volcanic origin. It is located at around 200 kilometers in front of Sahara’s coast in the northwestern Africa (Figure 1). Their climate is influenced by its geographical location, within the area of action of the steady trade winds belt, ruled by the high-pressure Azores cell and the low-pressure Icelandic cell, which shows a seasonal pattern throughout the year. During summer, these winds blow on a moderate way from N-NNE (frequencies between 90% and 95%), whilst in winter blow with lower intensity from NE (frequency over 50%) (Pérez et al., 2014). This climate system makes them ideal for tourists, who arrive in large quantities every year. Taking into account the above cited atmospheric conditions it is natural that wave conditions in the archipelago are generally mild or moderate, with two northern extreme sides (west and east) of the islands seen the most energetic ones, mainly during winter with a clear seasonal pattern (Guerra & Rodríguez, 2021). Furthermore, tides are mesotidal, with a semidiurnal pattern, oscillating between 0.5 m and 3 m, with an average value of about 1.5 m (Di Paola et al., 2020).
From a geographical point of view, there is a marked difference between the western and eastern islands, the western side islands (La Gomera, El Hierro, Tenerife, and La Palma) are the youngest and the highest ones. In contrast, the eastern islands (Gran Canaria, Lanzarote, La Graciosa, and Fuerteventura) are the older islands with mostly flat landscapes (Masson et al., 2002; Stillman, 1999). Something similar happens with the bathymetry, the western side is deeper, reaching 3 thousand meters between La Gomera, El Hierro, and La Palma islands, on the contrary on the eastern side of Fuerteventura, Lanzarote and La Graciosa maximum depth only reaches 1 thousand meters. Furthermore, politically and administratively the islands are divided into two provinces: Santa Cruz de Tenerife and Las Palmas (See Figure 1). Likewise, each province has its own maritime captaincy, which have jurisdiction over their respective waters (J. Hernández, 2018). The present work focuses on passengers and cargo maritime traffic disruptions to or from ports located in Las Palmas province due to bad weather conditions.
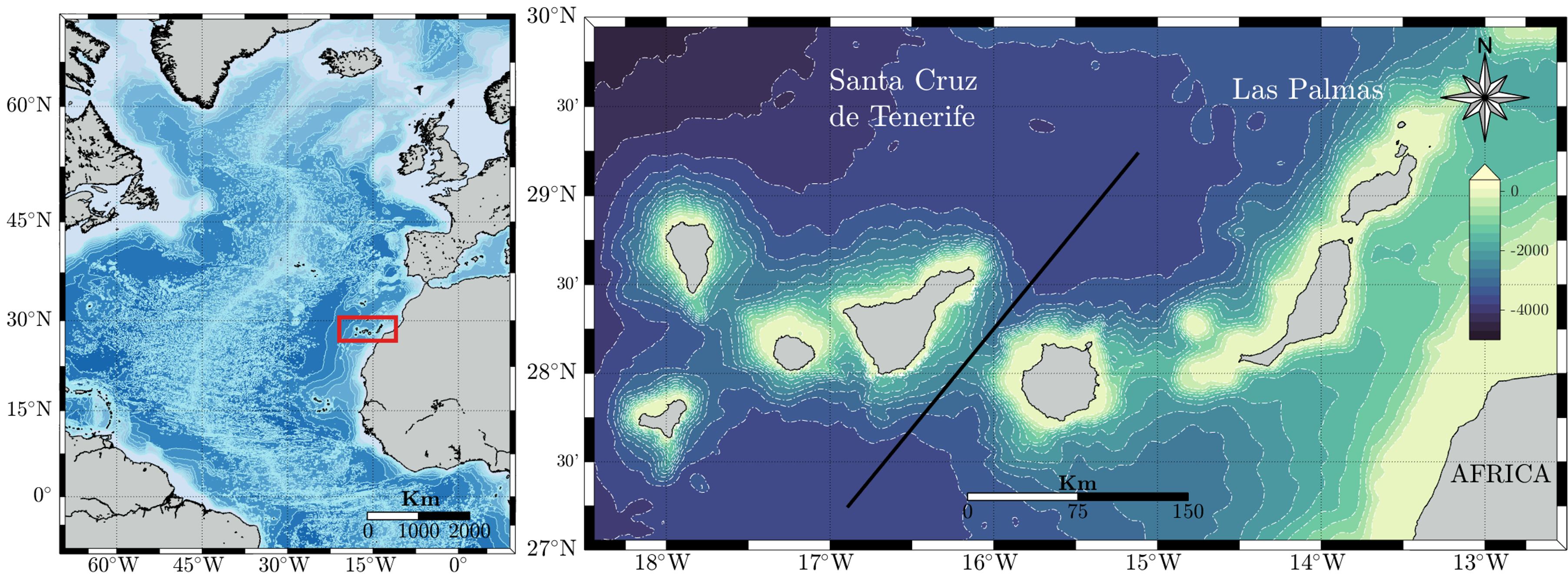
Figure 3.1: Location of Canary Islands (left). Zoom of the Canary Islands with a separation line between the two provinces: Santa Cruz de Tenerife and Las Palmas (right)
3.2 Data
The present study is based on various information sources of uneven nature. Thus, information regarding goods and passengers’ transportation between islands has been provided by the Spanish Ports institutions. Economic and demographic statistics have been obtained from the Canarian Institute of Statistics (ISTAC), these datasets have a cutoff in 2019, since after COVID-19 the economy collapsed, changing the number of regular routes. Information on routes among the islands corresponds to the four shipping companies transporting passengers and goods within the archipelago. Meteoceanic data are provided by Puertos del Estado and are derived by means of a hindcasting approach, which consists of two main steps. First, wind conditions over the area of interest are reproduced by means of a numerical model on the basis of past atmospheric analyses. Then, this information is used as input to a third generation wave numerical model, such as SWAN (Booij et al., 1999), to reproduce the corresponding wave conditions at multiple nodes of a numerical grid. In this case, nodes are named as SIMAR points and in most cases the associated datasets cover the period from 1958 to the present but in some cases, these extend from 2012 up to date, as a generation model. SIMAR points were selected to adequately reproduce meteoceanic conditions along the different maritime routes used by regular shipping companies that travel from or to the islands of Las Palmas province. Their location and nomenclature used to refer to each of them is shown in Figure 2.
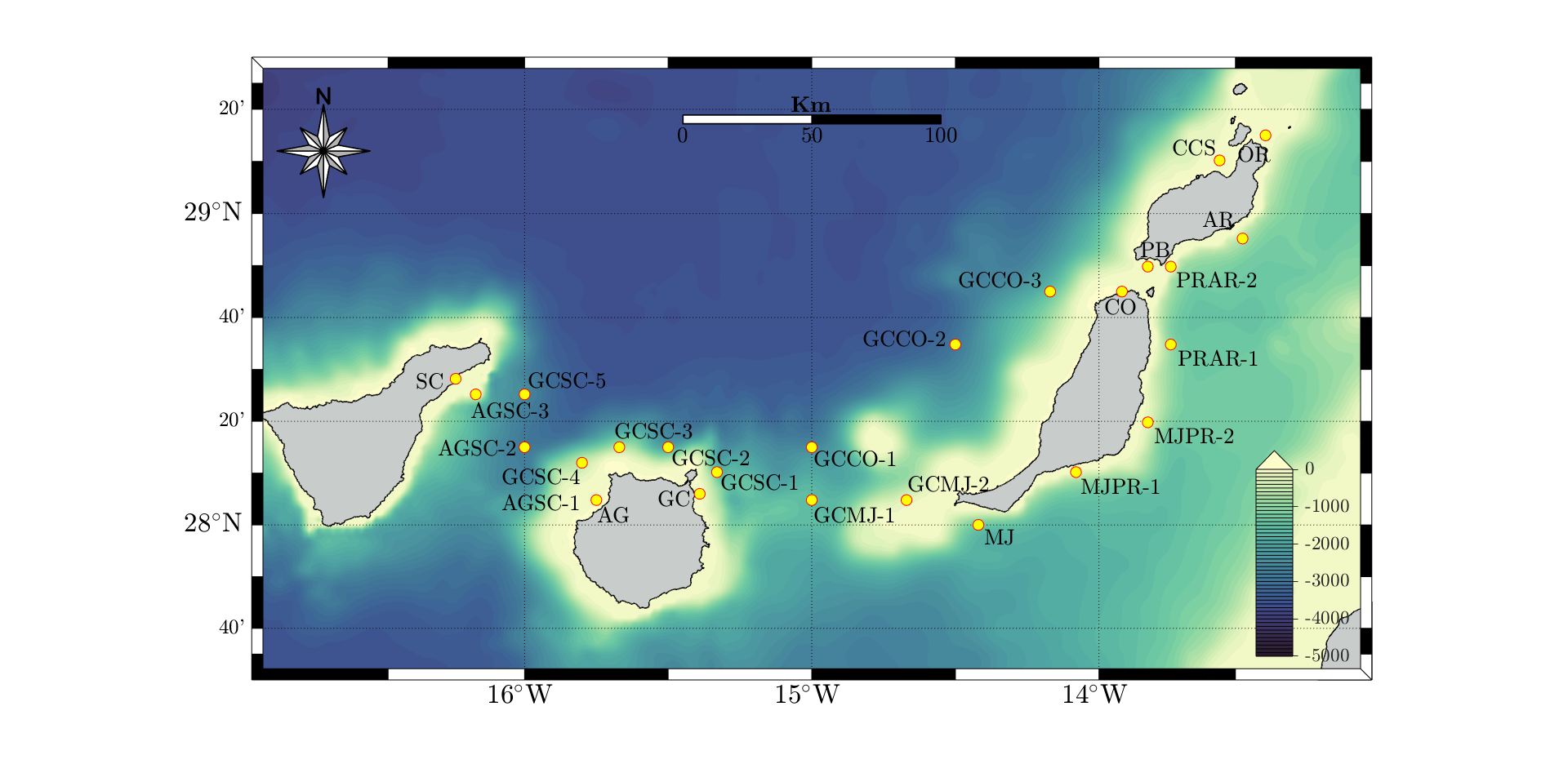
Figure 3.2: Hindcasting points location and nomenclature.
It is noteworthy that information provided by numerical models does not come from instrumental measurements and therefore have a considerable degree of uncertainty (Abdolali et al., 2021). However, the employ of numerical prediction models is a common practice due to the lack of long-term reliable data. Accordingly, whenever possible, validation against experimental data should be carried out. In this sense, it should be noted that meteoceanic information used in this study has been checked in previous studies with adequate results in general (e.g. Gonçalves et al., 2014; Sierra et al., 2013) but some limitations mainly due to the complexity of the islands’ geometry and orography (Guerra & Rodríguez, 2021). Accordingly, this uncertainty needs to be taken into account when analyzing the results.
The final product provided by the wave hindcasting approach is the directional spectral density function, \(S(f, θ)\), which represents the energy contribution of any wave component to the measured wave field in terms of the frequency, f, and propagation direction, \(θ\). Sea state parameters obtained for each SIMAR point, have been derived from spectral moments of the directional spectral density function, \(S(f, θ)\). The central parameter to characterize sea state is the significant wave height \((H_{m0})\), defined as four times the square root of the zero-th order spectral moment, \(m_{0}\), being the total energy of the process.
\[\begin{equation} H_{m0}= 4 \sqrt{(m_{0})} \tag{3.1} \end{equation}\]Regarding wave periods, two of the most common parameters used are the average wave period \((T_{02})\), defined in terms of spectral moment as
\[\begin{equation} T_{02} = (\frac{m_{0}}{m_{2}})^(1/2) \tag{3.1} \end{equation}\]The other one is the spectral peak period \((T_{p})\), which is the period associated with the most energetic spectral wave components. \(T_{p}\) is defined as the inverse of frequency at which the spectral density function attains its maximum \((f_{p})\).
\[\begin{equation} T_{p}= \frac{1}{f_{p}} \tag{3.1} \end{equation}\]In contrast to \(T_{02}\), \(T_{p}\) is not calculated by means of spectral moments but regarding a single spectral estimate and therefore displays high statistical variability (Rodríguez et al., 1999). In relation to directional properties of wave fields, one of the most common parameter used to characterize their directionality is the mean spectral direction, \(θ_{m}\), (Vega & Rodríguez, 2007) which is defined as the mean direction over all the frequency bands in the two-dimensional spectrum \(S(f, θ)\). Another important parameter in the study of ship dynamics is the significant wave steepness. For a monochromatic wave train wave steepness parameter is defined as the ratio of wave height \((H)\) and wavelength \((L)\). That is,
\[\begin{equation} S= \frac{H}{L} \tag{3.1} \end{equation}\]Assuming linear wave theory, the dispersion relationship allows to express wavelength in deep waters as
\[\begin{equation} L= \frac{gT^{2}}{2 \pi} \tag{3.1} \end{equation}\]Considering a wave train which can be characterized by the significant wave height, H_m0, and the peak period, T_p, wavelength can be expressed as
\[\begin{equation} L_{p}= \frac{gT_{p}^{2}}{2 \pi} \tag{3.1} \end{equation}\]So that significant wave steepness in terms of the peak period is given by
\[\begin{equation} S_{p} = \frac{H_{m0}}{L_{p}} =(\frac{2 \pi}{g}) \frac{H_{m0}}{T_{p}^{2}} \tag{3.1} \end{equation}\]For further information these and other wave parameters the reader is referred to general texts, such as the one by Massel, 1996. Information on the disruption of maritime lines has been obtained from two main sources. First, that provided by Fred Olsen Express, covering the period from 2017 to 2020. On the other hand, that retrieved from the JABLE database, which is a compilation of local press created by University of Las Palmas de Gran Canaria, containing news from 1808 to the present. Regarding this kind of information, despite its usefulness in the absence of more reliable information, it is necessary to indicate that it can be biased and incomplete, due to diverse motives, such as readers preferences, showing them what they consider of interest. Thus, a significant number events (suspensions, delays, or diversions of inter-island maritime regular traffic due to bad weather conditions) identified from the first source are not covered by the press. However, this source has the advantage of covering a long period of time and including information on events with the greatest socioeconomic impact. Finally, the development of a preliminary economic assessment, considering only the tourism sector, is based on data provided by (ISTAC, 2021).